The PhD supervisors and projects for fully funded studentships beginning in October 2024 are listed below. Applications for these IPPP studentships are also considered in the particle theory division of the math department, under the rubric of the Centre for Particle Theory. See here for information on how to apply, and indicate interest in physics in the application materials if it is the projects below which are your main interest.
Neutrino Physics
Particle physics and cosmology are entering an exciting era. Recent advancements in neutrino and gravitational wave experiments provide valuable insights into particles at the smallest scales and the universe at the earliest times. However, there is a need for improved precision in calculations and a more rigorous approach to comparing physics models with data.
This PhD vacancy offers several research projects:
- Exploring Neutrino Properties: This project utilizes the latest astrophysical data to investigate the properties of neutrinos, including their mass, lifetimes, and interactions.
- Investigating Non-Standard Cosmologies: This project explores the relationship between non-standard cosmologies, such as a universe dominated by primordial black holes, and theories that explain small neutrino masses, the matter-antimatter asymmetry, dark matter, and dark radiation.
- Using Gravitational Wave and Neutrino Data: This project assesses the viability of Grand Unification and neutrino mass models by leveraging the complementarity between gravitational wave and neutrino data.
These projects encompass a range of research, from more practical and data-driven work to the more theoretical in nature.
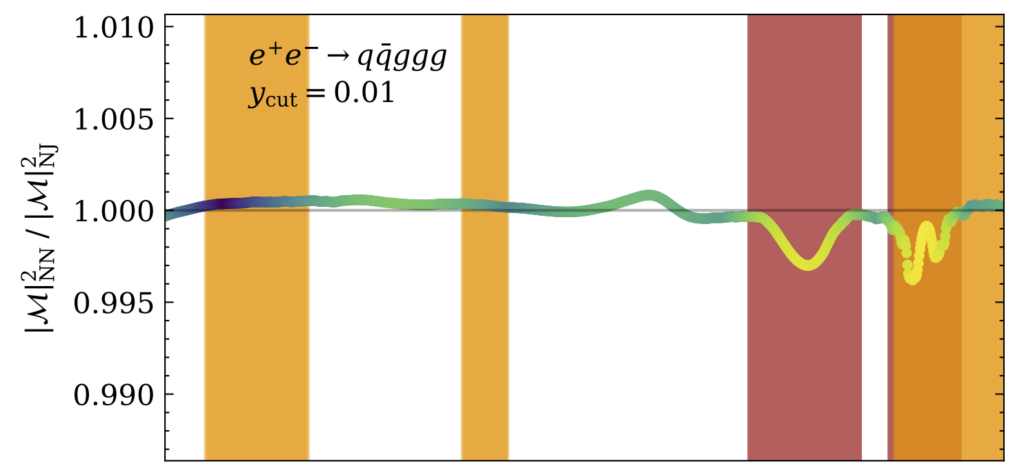
Precision calculations for present and future colliders
After the successful completion of Run I and II of the Large Hadron Collider (LHC) the much anticipated discovery of signals of beyond-the-Standard-Model physics is still lacking. Precision tests scrutinising the Standard Model are of prime importance in its physics programme, now and in the foreseeable future. At the same time new physics searches are looking for increasingly small signals demanding more precise estimates of the Standard Model backgrounds. Run III, having commenced recently, and the High-Luminosity upgrade thereafter, will further increase the available luminosity, enlarging the statistical prowess of the recorded data in most physics regions of interest. This expansion of sensitivity in both precision measurements and new physics searches in the multi-TeV region necessitates an immense improvement of the accuracy of theoretical predictions.
Further, strongly interacting new physics signals have been largely excluded by now, putting an emphasis on weakly interacting models and their electroweak Standard Model backgrounds. This is not only true for ongoing precision LHC measurements, but all the more so for all proposed future e+e− colliders. Lacking the vast energy range of a hadron collider they focus, in one form or another, on measurements with unprecedented precision to probe the Standard Model and, possibly, expose any deviations from its predictions.
The research performed in this PhD aims to provide precision calculations for relevant processes and observables at the LHC and future colliders that are necessary to contrast and test the predictions of our current best theory, the Standard Model, against the expected high-precision experimental data. In consequence, contribution from new physics not contained in the Standard Model to such processes and observables can be analysed and interpreted either as discoveries or more and more stringent exclusion limits.
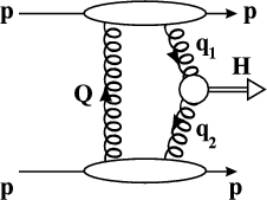
Theories to enable searches for new physics with high energy and high precision experiments
The research performed in this PhD position seeks to deepen our understanding of the fundamental theories that describe our universe. Theories addressing the open problems not explained within the Standard Model of particle physics, for example the question what constitutes dark matter, how neutrinos obtain their masses, or whether new physics can explain the difference between the matter and antimatter we observe in the Universe, or how we can observe the cosmic neutrino background, have direct and indirect effects. In parallel novel experimental technologies have changed the way we can discover new physics significantly. We now have high-statistics available to probe the properties of the Higgs boson, gravitational wave detectors have enabled to search for completely new signals, and quantum sensors have made enormous progress in the last decade. The goal of this research is to consistently describe theories of new physics taking into account all effects dictated by the mathematical consistency and the symmetries of the theory, to explore the implications of this description and to advise novel ways top observe these direct and indirect effects. Special focus will be on fully exploiting the experimental advances both in high-energy physics and high-precision physics. Familiarity with both quantum field theory calculations and scientific software are beneficial for this project.
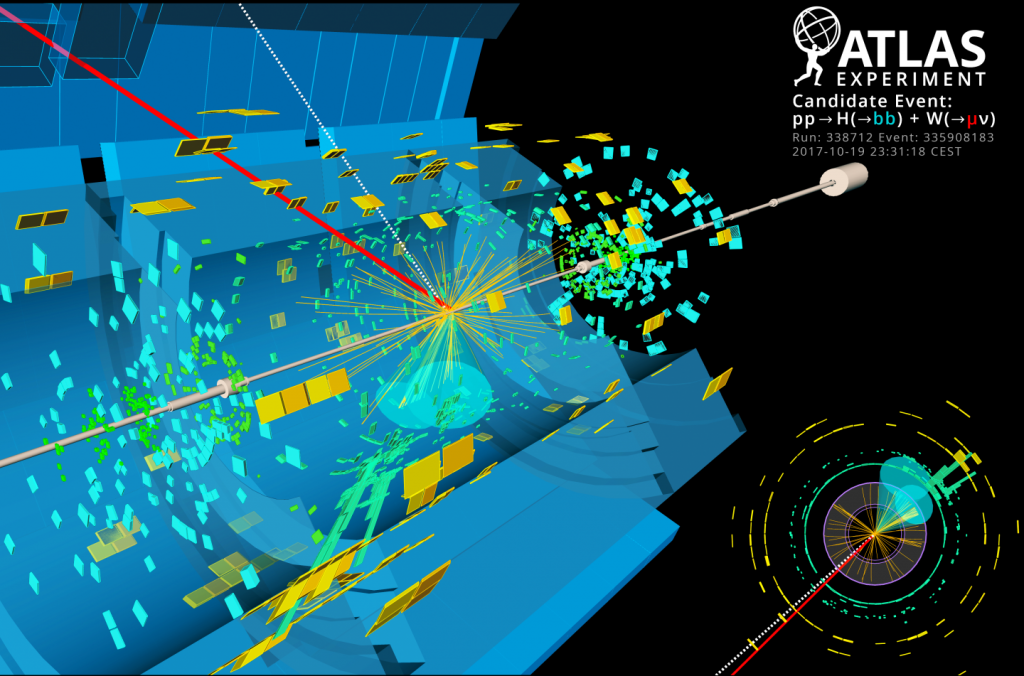
Unveiling the Neutrino Mysteries: From Particle Physics to Astrophysics
Despite the successes of the Standard Model (SM), it is widely acknowledged that it cannot be the ultimate theory of Nature due to various theoretical constraints and observational challenges. One of these challenges is the lack of explanation for the mechanism behind neutrino masses, indicating that New Physics phenomena are likely at play in the neutrino sector.
The discovery that neutrinos are massive particles originated from observing flavor transitions. Studied across various energies and baselines, recent results highlight less constrained parameters: mass ordering and the CP-violation phase. This project aims to test the neutrino oscillation paradigm using data from current and upcoming experiments.
New neutrino species, known as “sterile neutrinos,” with masses at the eV scale, have been proposed to explain anomalies such as the excess events observed in the MiniBooNE and LSND experiments. Tensions detected in this scenario drive the exploration of alternative scenarios to address these discrepancies. At higher mass scales, a sterile neutrino could potentially account for the origin of neutrino mass. With upcoming measurements, we will actively search for signals that these states might leave in the detectors.
Besides these measurements, astrophysical sources offer a valuable setting to explore neutrino properties. This project will examine neutrinos emitted by diverse astrophysical sources, ranging from MeV neutrinos emitted by stars to the TeV-scale neutrinos detected from recent sources identified by IceCube and even the predicted EeV-scale cosmogenic neutrino flux. The wide energy range and extensive distances traveled by these neutrinos provide an ideal opportunity to test the Standard Model and understand the Universe across varying energy scales.
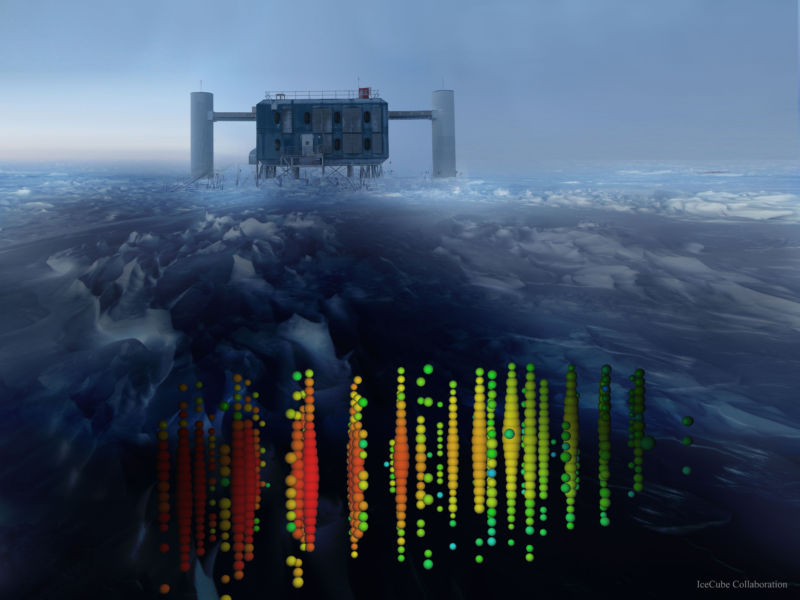
Phenomenology at Particle Colliders and Simulations
High-energy collisions at particle accelerators continue to provide us with the best and most varied data and insights concerning the fundamental constituents of matter and the rules according to which they interact. After the discovery of the Higgs boson a decade ago, the current flag-ship experiments at the CERN LHC are entering a phase where their ever increasing precision is cornering and excluding ideas for extensions of the Standard Model. At the same time, the particle community is discussing the experiments after the LHC era, with a wide array of options and a broad range of physics opportunities.
For all these facilities at current and future particle colliders simulations bridge the gap between theoretical concepts and experimental data, turning the former into calculations and results that can be directly compared with the latter. Consequently, simulation tools such as SHERPA event generator are a unique and non-dispensable part of all phenomenological efforts to shed light on the behaviour of matter at its most fundamental level.
Frank’s PhD project will center around extending the capabilities of SHERPA with the latest theoretical results and methods and using the event generator to propose new measurements to the experimental community.
Shedding light on dark matter with new phenomenological tools and astrophysical data
H The particle nature of dark matter, constituting greater than 80% of the matter content of the Universe, continues to elude us. Recent breakthroughs in astronomy may hold the potential to unravel this mystery, as they allow access to previously concealed regimes of the cosmos.
In particular, wo novel types of astrophysical data may lead to a breakthrough in our understanding of dark matter. Firstly, there is the advent of gravitational wave (GW) astronomy, marked by the detection of a binary black hole merger by the LIGO/Virgo collaboration in 2015. In 2023, pulsar timing arrays also reported a signal consistent with nanoHz gravitational waves. Future observation runs and future GW experiments will advance significantly in frequency range and sensitivity, opening up new directions for phenomenological studies of particle physics. Secondly, infrared (IR) astronomy is poised to revolutionise our understanding of the cosmos. With the launch of next-generation space telescopes including JWST and the Nancy Grace Roman Space Telescope (RST), and the refinement of ground-based observatories, we will be able to probe deeper into the universe than ever before, capturing images and spectra of celestial objects that remain elusive in other wavelengths.
In this PhD project you will work on phenomenology of dark matter through the interpretation of astrophysical data, paving the way to answer fundamental questions about the nature of dark matter. You will work with a sophisticated arsenal of tools, which will likely include stellar evolution simulations and various data analysis and machine learning techniques, and collaborate with experts across different fields.